The
process called convection is easily observed in ordinary experience, but
has been greatly misunderstood in the geosciences for decades, being
widely assumed to take place in the Earth's fluid core. But convection
in the Earth's core, as discovered by J. Marvin Herndon, pictured at
left, is physically impossible.
Heat a pot of water on the stovetop. Before it starts to
boil, the water begins to circulate from bottom to top and from top to
bottom. This is called convection and it can be better observed by
adding a few tea leaves, coffee grounds, celery seeds, or the like,
which are carried along by the circulation of water. Convection occurs
because heat at the bottom causes the water to expand a bit, becoming
lighter, less dense, than the cooler water at the top. The warmer, less
dense, water rises to the top as the cooler, denser, water descends.
This all seems so simple that it is no wonder that the convection
process has been widely (but falsely) assumed to occur deep within the
Earth’s core.
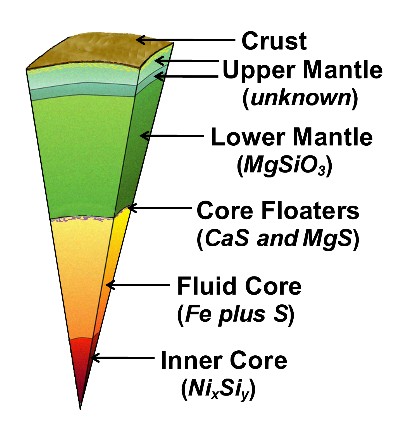
About 95% of the mass of the Earth consists of just two
parts; the fluid, iron-alloy core and the solid, silicate-rock mantle,
as illustrated at right. In 1939, Walter Elsasser postulated Earth-core
convection to make tenable his idea that the Earth’s magnetic field is
generated by convection-driven dynamo action within the Earth’s fluid
core [1]. At the time, and until recently, there was no reason to
suppose that any fluid, electrically conducting region, except the main
core, exists within the Earth [2, 3]. Note that Earth-core convection
was not independently observed, but rather, was assumed to exist so as
to satisfy the underlying conditions for a different theory.
Subrahmanyan
Chandrasekhar [4], pictured at left, described convection in the
following way: “The simplest example of thermally induced convection
arises when a horizontal layer of fluid is heated from below and an
adverse temperature gradient [i.e., the top is cooler than
the bottom] is maintained. The adjective ‘adverse’ is used to qualify
the prevailing temperature gradient, since, on account of thermal
expansion, the fluid at the bottom becomes lighter than the fluid at the
top; and this is a top-heavy arrangement which is potentially unstable.
Under these circumstances the fluid will try to redistribute itself to
redress this weakness in its arrangement. This is how thermal convection
originates: It represents the efforts of the fluid to restore to itself
some degree of stability.”
In 1900, Bénard observed the formation of a pattern of
cells [convection cells] developing in a thin layer of water heated from
beneath [5]. In 1916, Lord Rayleigh [6] derived a dimensionless number –
now called the Rayleigh Number – to quantify the onset of instability,
which would lead to convection in a thin, horizontal layer of fluid
heated from beneath. For decades, calculation of a high Rayleigh Number
has been taken to justify the existence of Earth-core convection. The
advice for students, generally speaking, as stated in Herndon's book,
Maverick’s Earth and Universe [7] is to “Look deeper and look
questioningly.” And, certainly, that is the case here.
What
seems to have been overlooked is that the Rayleigh Number was derived
from assumptions that are inconsistent with the physical parameters of
the Earth’s core. Rayleigh assumed an “incompressible” fluid,
i.e.,
a fluid of “constant” density throughout, except as modified by thermal
expansion at the base, and pressure being “unimportant” (quotes from
Lord Rayleigh [6]). The Earth’s core is not “incompressible”, but
consists of a compressible fluid which is, in fact, compressed by the
weight of the mantle and crust above and by its own weight. The Earth’s
core is not of “constant” density; its base is about 23% more dense than
its top due to the pressure of the weight above [8], illustrated in the
figure at right. Thus, the dimensionless Rayleigh Number is an
inappropriate indicator of convection in the Earth’s core.
It is instructive to consider and to discuss some of the
reasons why convection, as commonly observed on the stovetop and as
described above by Nobel Laureate Chandrasekhar, is impossible within
the Earth’s core. On the stovetop, convection occurs because heat at the
bottom causes the water to expand a bit [much less than 1%], becoming
lighter, less dense, than the cooler water at the top. This is a
potentially unstable, top-heavy arrangement which the fluid attempts to
redress by convection. So, in what ways is that different from the
Earth’s core and how do those differences impact the convection process?
The Earth’s core differs from the stovetop example in two
important ways. First, as shown in the figure at right, because of the
over-burden weight, the Earth’s core is about 23% more dense at the
bottom than at the top [8], as illustrated at right. The tiny, tiny
amount of thermal expansion at the bottom cannot make the Earth’s core
top-heavy and cannot cause a thermally-expanded “parcel” from the bottom
to float to the top of the core as required for convection. Thus, the
Earth’s core cannot engage in convection. Second, because the Earth’s
core is wrapped in a thermally insulating blanket, the silicate-rock
mantle, heat cannot be efficiently removed from the top of the core. So,
maintaining an “adverse temperature gradient” [i.e., the top of
the core being cooler than the bottom] for extended periods of time, a
condition necessary for convection, is impossible [3].
What
is the main implication of no Earth-core convection? From the standpoint
of geomagnetic field generation, the implication is quite clear: Either
the geomagnetic field is generated by a process other than the
convection-driven dynamo-mechanism, or there exists another fluid region
within the deep-interior of Earth which can sustain convection for
extended periods of time. Herndon has provided the reasonable basis to
expect long-term, stable convection in the georeactor sub-shell, and
have proposed that the geomagnetic field is generated therein by the
convection-driven dynamo mechanism [3, 9-11]. The figure at left is a
schematic representation of the interior of the Earth showing Herndon’s
georeactor. Heat produced by the georeactor nuclear sub-core is expected
to cause convection in the georeactor sub-shell. Heat brought to the top
of the sub-shell is expected to be transported away by the
thermally-conducting inner core heat sink which is surrounded by an even
more massive thermally-conducting fluid core heat sink.
For Teachers:
Teaching Students to
Question Earth-Core Convection (click here for pdf)
YouTube Demonstration:
Origin of Earth's
Magnetic Field (click here)
Return to Home Page NuclearPlanet.com
|